Monday, 29 June 2020
California's Current and Former Structural Ordinances
Monday, 22 June 2020
Lessons from the ASCE 41 Basic Checklist
Ms. Kelly is an experienced structural engineer with a focus on seismic risk. She has extensive experience in structural failure investigations, seismic structural design, and seismic risk assessments. Through the School of P.E., she has taught a 32-hour course for the California Seismic P.E. Exam, authored several blog posts, and contributed to other review products. She has a Bachelor of Science in Civil Engineering from Johns Hopkins University and a Masters of Engineering in Structural Engineering from Lehigh University.
Monday, 15 June 2020
How is ASCE 7 Organized for Seismic Engineering
Ms. Kelly is an experienced structural engineer with a focus on seismic risk. She has extensive experience in structural failure investigations, seismic structural design, and seismic risk assessments. Through the School of P.E., she has taught a 32-hour course for the California Seismic P.E. Exam, authored several blog posts, and contributed to other review products. She has a Bachelor of Science in Civil Engineering from Johns Hopkins University and a Masters of Engineering in Structural Engineering from Lehigh University.
Monday, 8 June 2020
Discussion on Building Frame vs. Bearing Wall Systems
Ms. Kelly is an experienced structural engineer with a focus on seismic risk. She has extensive experience in structural failure investigations, seismic structural design, and seismic risk assessments. Through the School of P.E., she has taught a 32-hour course for the California Seismic P.E. Exam, authored several blog posts, and contributed to other review products. She has a Bachelor of Science in Civil Engineering from Johns Hopkins University and a Masters of Engineering in Structural Engineering from Lehigh University.
Monday, 1 June 2020
How Hard is the California Seismic P.E.?
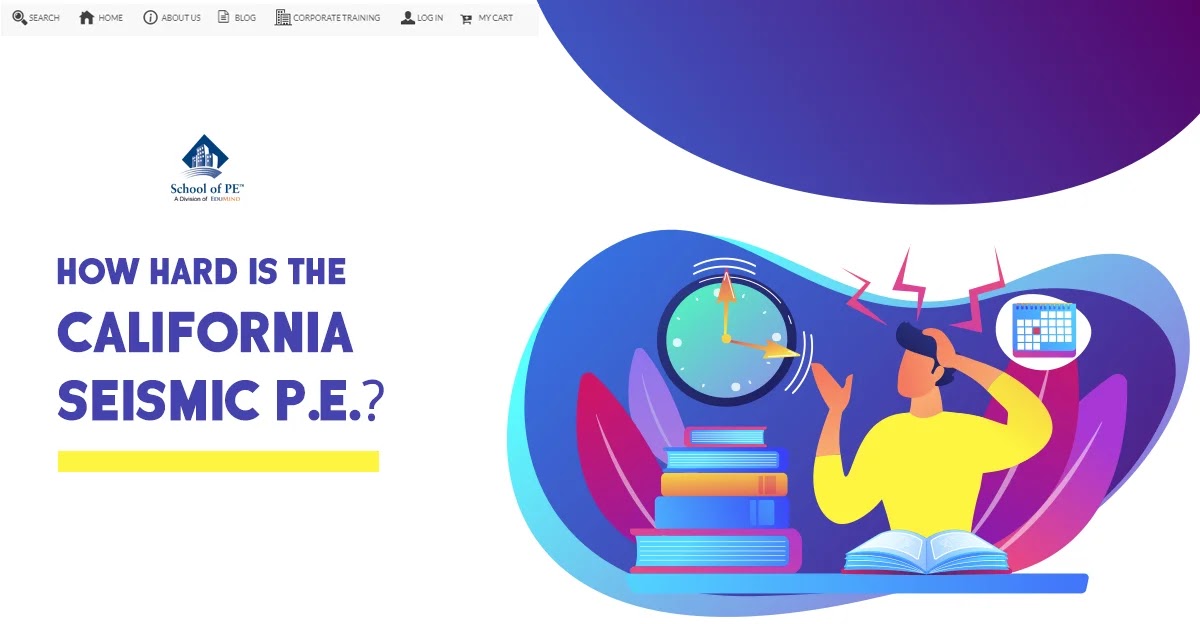
Ms. Kelly is an experienced structural engineer with a focus on seismic risk. She has extensive experience in structural failure investigations, seismic structural design, and seismic risk assessments. Through the School of P.E., she has taught a 32-hour course for the California Seismic P.E. Exam, authored several blog posts, and contributed to other review products. She has a Bachelor of Science in Civil Engineering from Johns Hopkins University and a Masters of Engineering in Structural Engineering from Lehigh University.